In 1954, Atomic Energy Chairman Lewis Strauss promised energy "too cheap to meter." In 2025, we interviewed 15 pioneers working to shape the future of energy. Our second frontier film is out now: 'TOO CHEAP TO METER' — their vision may finally deliver on that promise.
With this release from the Frontier Film series, we commissioned Andrew Miller to write an accompanying essay for the film. The essay expounds on some of the idea highlighted in the film, giving greater context and data as both an aid for viewers and guide for further exploration.
We suggest that you first watch the film, then come back to read through this essay.
Written by Andrew Miller | Edited by Niko McCarty | Directed by Jason Carman
“Too cheap to meter.”
This was a bold promise about nuclear power, that it would make electricity so abundant we wouldn't bother to track or itemize its use.
That promise wasn’t kept. But we remember it, because the vision it offered was so powerful: of a world where we unlocked all the promise of abundant energy.
Energy isn't just a feature of civilization. It’s not even just an important feature of civilization. It’s the fundamental force that creates, sustains, and advances civilization.
Without plentiful energy, modern society collapses. But if energy is even more plentiful, human potential expands exponentially. The connection is direct and causal: every significant leap in human progress throughout history has been powered by accessing more energy-dense resources. From wood to coal, and then to oil, and then to nuclear, each transition enabled exponential growth in human capabilities.
Energy doesn't just power our devices; it fabricates everything around us. Consider a smartphone: it requires energy to charge its battery. But it also required energy to mine the aluminum and the rare earth elements that make up its body; to smelt these into usable forms; to manufacture these into its case and its chips; and to assemble them together. Each step in the supply chain, from simple to complex, depended on energy, in vast quantities and precisely deployed.
Most people intuitively recognize the importance of energy to industrial civilization. Steel mills need electricity, and airplanes need fuel. But energy undergirds everything, from the concrete that makes our structures to the fertilizer that feeds billions.
One reason this connection is easy to miss is that the energy-progress connection may seem to have weakened recently. In the West, energy use per person has plateaued while the economy has continued to grow.
That apparent weakening has led some to believe we can achieve greater and greater prosperity without more sources of energy; that efficiency alone will save us.
This comforting narrative ignores two realities: efficiency has fundamental limits, and billions still live without enough energy. Addressing humanity’s greatest challenges—from climate change to global poverty—requires us to develop energy sources that are vastly more plentiful than what we have today.
Story Company's second documentary film in their Frontier Films series, Too Cheap to Meter, challenges us to finally make the promise in its title come true. There are a variety of paths to follow, but if we take them, we will have the critical resource that will help us to solve our biggest problems. And more than that, it will allow us to build new things that for now we can only dream of: fresh water for all, the repair of our damaged climate, and even a multi-planetary civilization.
That is what energy abundance offers. It is the next great human enterprise.
The Energy-Density Imperative
Today, we stand on the brink of breakthroughs in nuclear power, fusion research (the process of combining atomic nuclei to release energy), and other innovative technologies. These new approaches could preserve a comfortable, sustainable Earth while also enabling humanity to explore realms that we barely imagine. If we understand why such advances are necessary, we can accelerate their development and adoption.
The growth of human civilization is directly correlated with—indeed, has been caused by—our ability to harness increasingly dense sources of energy. Each time humanity has been able to successfully exploit a new, denser energy source, we have unlocked new capabilities, which in turn allowed leaps forward in human development.
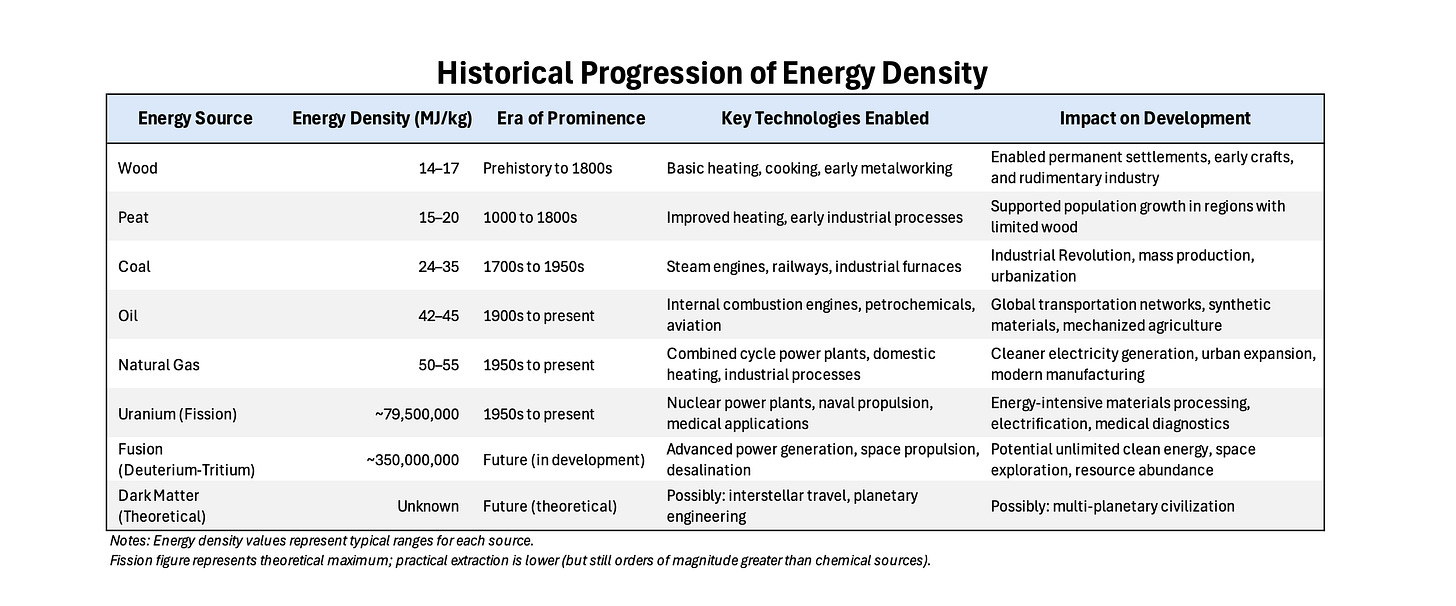
The progression in energy densities is striking. The leap from wood to coal was a rough doubling in energy density. The most dramatic leap in energy density came with nuclear fission, where uranium in conventional reactors provides more than 10,000 times the energy per unit mass than any fossil fuel. Looking forward, fusion promises even greater density at around 350 million MJ/kg, approaching the theoretical maximum energy conversion possible.
Each transition in energy density also corresponded with specific, tangible advances in civilization. The shift from wood to coal enabled the mechanization of industry, powering steam engines that transformed manufacturing and transportation. Coal's concentrated energy made possible the first railways and factories that operated continuously, freeing production from the limitations of human and animal muscle power.
When oil superseded coal as our primary energy source, its superior energy density and liquid state revolutionized transportation. Automobiles, aircraft, and modern shipping became viable, dramatically shrinking distances and accelerating global commerce. The petroleum revolution extended beyond transport: it provided feedstock for plastics, fertilizers, and countless materials that define modern life. As Isaiah Taylor, founder of Valar Atomics, notes in Too Cheap to Meter, “If you look around any room, most of what you're going to look at” is “built out of carbon,” derived from petroleum distillates.
Scientists and engineers in the nuclear age unlocked entirely new orders of magnitude in energy density. A single kilogram of uranium fuel can produce as much energy as 1,500 tons of coal. This extraordinary density enabled consistent, reliable electricity generation at unprecedented scale, powering the electrification that transformed societies from the 1970s onward.
Examples include France and Japan’s embrace of nuclear energy on a national scale after the 1973 oil crisis, which permitted the emergence of new industries: aluminum smelting in France, electronics production in Japan, and high-speed, electrified intercity rail in both countries. In the United States, as Doug Bernauer, the CEO of Radiant Industries, observes in Too Cheap to Meter, “when nuclear technology was invented and we started to scale it up... we stopped burning oil, broadly, as a nation, in large scale plants” to generate electricity. “We just didn't have to do that anymore.”
The relationship between energy density and civilization advancement demonstrates a critical principle: each leap in energy density provides not merely a quantitative improvement but a qualitative transformation in what becomes possible. The compounding effects cascade throughout society:
Agricultural productivity: The Haber-Bosch process for nitrogen fixation, dependent on dense energy inputs, has expanded agricultural capacity enormously, supporting billions of additional human lives. This effect is easy to miss, but arguably is the most important on this list.
Industrial capacity: Denser energy enables new industrial processes. Some of these are older processes but made more intense, like aluminum smelting, which requires ~15MWh of electricity per ton. Others are wholly new, like semiconductor fabrication, which rely not only on high-powered equipment like laser lithographers and etchers, but also on production environments featuring precisely controlled cleanrooms and temperatures. In practice, such facilities need fifty-to-one-hundred times more energy per square foot than contemporary office buildings.
Transportation range and speed: Higher energy density directly translates to greater mobility. The progression from sail to steam to internal combustion to jet engines mirrors the progression in energy density. Early steamships, running on coal, could travel 5 miles per hour at sea; gasoline, with its higher energy density, permits cars today to comfortably travel 85 miles per hour on roads, while the Boeing 747 can travel at 570 miles per hour in the air.
Information technology: The digital revolution depends on reliable, clean electricity, predominantly from high-density sources that can provide consistent baseload power; some estimates say that the global Internet consumes ~200 to ~250 terawatt-hours of electricity each year, or about 1% of all electricity used in the world. Training large AI models is particularly demanding of energy, measured in the hundreds of thousands of kilowatt-hours.
Medical advances: From medical imaging to sterilization systems, modern healthcare relies on energy-intensive technologies made feasible by dense power sources. Examples include MRI machines, which require superconducting magnets and consequently have to be maintained at temperatures measuring only a few degrees Kelvin, or autoclaves, which sterilize surgical instruments, and must therefore maintain temperatures above 120 degrees Celsius.
This history is instructive. The most promising pathways for human advancement involve continued increases in energy density: through advanced nuclear fission, commercial fusion, or as-yet undeveloped technologies. The remarkable potential of fusion represents the next logical step in this progression. “The more that humans can consume energy, the more productive we become, the more prosperous we become, the more things we can invent, more people we can have on planet Earth,” says Brandon Sorbom, Co-Founder and Chief Scientific Officer of Commonwealth Fusion Systems, in Too Cheap to Meter. “By any metric that people use to track quality of life, it always gets better with energy.”
Efficiency and the Decoupling Phenomenon
The strongest argument against this view is the fact that, since the 1970s, economic growth appears to have decoupled from energy consumption. In many advanced economies, GDP has continued to rise steadily, even as per-capita energy use has plateaued or declined. Has the link between power and prosperity been broken?
At first glance, the data seems compelling. In the United States, primary energy consumption per capita peaked around 1979 at approximately 350 million BTU and has gently declined since, even as per-capita GDP more than doubled in real terms. Similar patterns emerge across OECD countries: the EU's energy intensity—energy consumed per unit of GDP—fell by 43% between 1980 and 2021, according to the International Monetary Fund. Particularly prosperous jurisdictions, like California and Denmark, have boasted economic growth this century while keeping energy consumption per capita at the same rates they had at the century’s beginning.
One might interpret this decoupling as proof that efficiency improvements allow us to maintain growth while using less energy.
If we look more closely, though, this story begins to fall apart.
The silent change, hiding in the statistics, is improvements in energy efficiency. These improvements have functioned as a ‘new source’ of energy; when we double the efficiency of a process, we double the output from the same energy input. Examples include LED lighting, which use 85% less energy than incandescent bulbs, or electric motors, which are three-to-five times more efficient than combustion engines for mechanical work. These improvements, and many others like them, have effectively multiplied the West’s economic output from each unit of energy consumed.
Efficiency is always a virtue, certainly, but there are limits to what can be gained from it. By definition, no process can exceed 100% efficiency. And practically, such a percentage is impossible to achieve, with more and more difficulty and expense required to raise efficiency levels less and less.
Even were it possible to reach 100%, though, there is still the problem of the Jevons Paradox: efficiency improvements in the use of a commodity tend not to lower that good’s consumption, but to increase it. Studying coal use in 19th century Britain, economist William Jevons noted that as factories became more efficient at their use of coal, they burned more of it. "It is wholly a confusion of ideas," he wrote, “to suppose that the economical use of fuel is equivalent to a diminished consumption. The very contrary is the truth.”
The pattern of behavior that Jevons identified has been consistently observed in industrial production since. From 1975 to the present, the average fuel economy of new vehicles in the United States has improved by approximately 105%, yet during this same period, average annual vehicle miles traveled increased by almost 2 trillion miles per year.
Lighting provides an even more dramatic example. The efficiency of lighting technology has improved about 1,000 times over since the early 19th century, yet over the same time, global per-capita consumption of artificial light has increased by roughly 10,000 times. In both cases, the effect is the same: greater efficiency made the activity less expensive, encouraging more of it.
Given this relationship, it is unsurprising that the nations today with the highest energy-efficiency standards, like Germany or Japan, remain among the world's highest per-capita energy consumers. Their efficiency enables greater economic output per unit of energy, but has not led to decreased absolute energy use. So efficiency improvements, while valuable, cannot substitute indefinitely for harnessing denser energy sources.
What the decoupling phenomenon shows, then, is not that energy is becoming less important to prosperity, but rather that we've found more sophisticated ways to employ it. Efficiency gains have indeed contributed to economic growth, functioning as a hidden form of energy abundance that statistical measures of raw consumption fail to capture. Yet these gains face inherent physical limits and, paradoxically, tend to increase rather than decrease total energy demand.
Energy efficiency, then, is valuable and useful, but ultimately is a complement to energy abundance rather than an alternative. Energy abundance must remain our goal—especially when we consider the billions still living in energy poverty, for whom efficiency does not offer a path to prosperity.
Energy Access and Global Development
The relationship between energy consumption and human well-being becomes most stark when we look at the world as a whole. Today, approximately 770 million people still lack access to electricity, while nearly 2.6 billion rely on traditional biomass—wood, charcoal, and agricultural residues—for cooking and heating. Globally, lack of access to abundant and high-quality energy is a profound limitation on human potential.
The scale, and enormity, of this inequality is vast. Annual per-capita electricity consumption ranges from less than 100 kilowatt-hours in the least developed countries to more than 12,000 kilowatt-hours in advanced economies; a 120X difference. Annually, in many parts of sub-Saharan Africa, an individual person consumes less electricity than does the average American refrigerator.
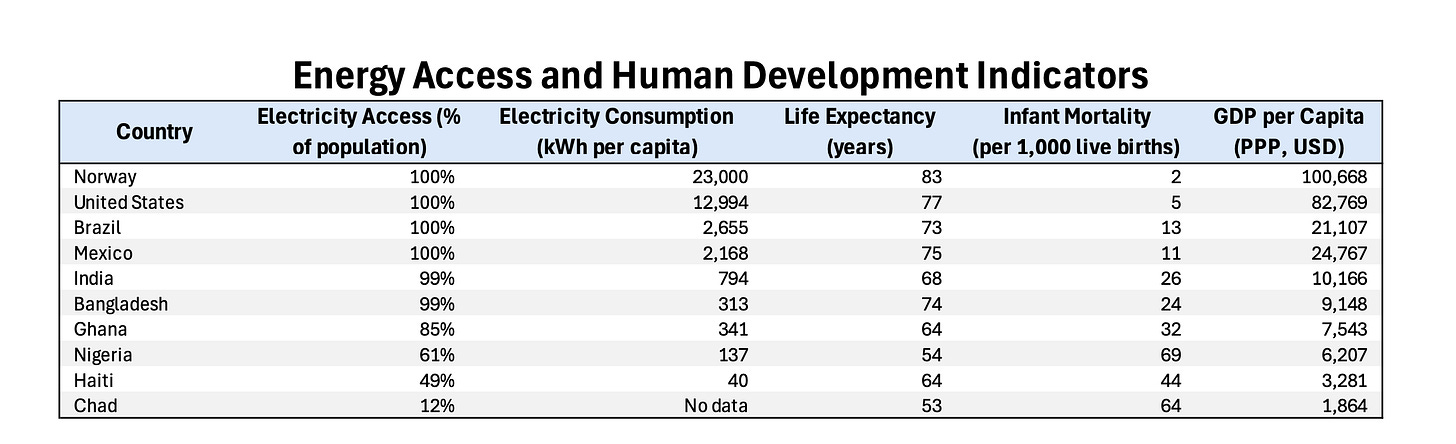
Energy poverty correlates strongly with reduced life expectancy, higher infant mortality, limited educational attainment, and restricted economic opportunity. As per the World Health Organization, approximately 3.8 million people die prematurely each year as a consequence of eating food cooked with biomass (burning these fuels indoors leads to poor air quality, which in turn leads to a variety of illnesses). In healthcare settings, lack of reliable electricity precludes refrigeration for vaccines, powered medical equipment, or lighting for nighttime care. Lack of energy hinders educational outcomes as well: without electric lighting, evening study time is reduced.
The development imperative is thus inseparable from the energy imperative. Bringing global energy consumption to even modest levels—half the per-capita use of developed nations—would require approximately doubling current global energy production. This reality highlights why efficiency alone, while necessary, cannot address global energy poverty. The magnitude of unmet need demands substantial expansion of energy production, worldwide.
Answering this need is important, but poses a further problem. Developing nations understandably prioritize expanding energy access over minimizing greenhouse-gas emissions. As the President of Senegal observed in 2022, “It is legitimate, fair, and equitable that Africa, the continent that pollutes the least and lags furthest behind in the industrialization process should exploit its available resources to provide basic energy… and achieve universal access to electricity.”
Given this reality, how can we avert global warming and mitigate the effects of climate change? If carbon-intensive options are the only ones available, then developing nations will take them, which means that other options must be provided. The ideal energy portfolio will combine multiple solutions. As Too Cheap to Meter shows, contemporary technologies, like solar with battery storage, and near-term ones, like small modular reactors, can (or could) provide cost-competitive, non-emitting power, as well as powering offsets for emitting sources.
There is a moral imperative to develop and scale these solutions as fast as possible.
Next-generation nuclear power represents one promising method of achieving this portfolio. Small modular reactors (SMRs)—nuclear facilities generating between 10 and 300 megawatts—offer advantages over the 20th century's gigawatt-scale plants. Ideally, SMRs will be manufactured in factories, transported to sites, and installed with significantly reduced capital costs and construction timeframes. Their modular nature would allow for incremental capacity additions, reducing financial risk and improving grid integration. For regions with unreliable grids or growing industrial bases, these could provide the consistent baseload power necessary for development without the emissions of coal or gas plants.
Meanwhile, in regions with limited infrastructure, solar photovoltaics have emerged as a transformative solution. Solar has experienced a 90% cost reduction over the past decade, with further declines anticipated as manufacturing scales and efficiency improves. Too Cheap to Meter highlights that solar deployment reached “600 gigawatts worldwide” in a recent year, “more than the total global nuclear fleet.” Its declining costs and modular nature make it particularly well-suited for rural electrification and distributed generation in developing regions, allowing communities to leapfrog traditional centralized grid infrastructure.
Fusion energy, if we can harness it, represents perhaps the ultimate energy source. Despite significant effort in the past, it has always proved elusive, but recent developments suggest achieving it may indeed be possible. As Future Energy discusses, for the first time, researchers have achieved fusion reactions that produce more energy than was directly used to initiate them. If progress continues, the potential of commercial fusion—still decades away—is unparalleled: abundant fuel from hydrogen isotopes, no risk of meltdown, minimal radioactive waste, and energy production at a scale that could transform civilization. As J.C. Btaiche, Founder and CEO of Fuse, states in Too Cheap to Meter, “Fusion will be the last energy source civilization will ever need.”
And there are other possibilities. Next-generation biofuels and synthetic fuels offer pathways to decarbonize sectors resistant to electrification, notably air travel, by providing a source of carbon-neutral, sustainable fuel. Advanced geothermal systems may provide continuous, dispatchable clean energy in many regions. Grid-scale energy storage continues to evolve, enabling greater integration of intermittent renewable sources.
Abundance vs. the Climate
The discussion thus far might suggest a simple dichotomy: ‘clean’ energy sources like solar, wind, and nuclear versus ‘unclean’ sources like coal, oil, and gas. Such a framing is intuitive, but misses a crucial insight from Too Cheap to Meter. The real divide is not between types of energy, but between sustainable and unsustainable energy systems.
Hydrocarbons, such as jet fuel, have inherent advantages that make them invaluable for certain applications. The energy density of jet fuel (approximately 43 MJ/kg) allows aircraft to carry sufficient energy for long-distance flight while maintaining reasonable weight. Batteries today, with their much lower energy density (<1 MJ/kg), come nowhere close.
The contemporary world relies on jet travel. If climate change was not an issue, it’s impossible to imagine that in the future we would fly less; indeed, we would fly more. What is required is a way to rely on these useful molecules, to an even greater extent, but in a way that offsets their impact on the atmosphere.
Several possibilities exist. One is to produce ‘sustainable aviation fuel’, by extracting carbon dioxide directly from the atmosphere, and combining it with hydrogen extracted with water to synthesize hydrocarbon fuels that are functionally carbon-neutral. When burned, these fuels release the same carbon that was recently captured, creating a closed cycle rather than adding new carbon to the atmosphere.
Such a process can be done today. The problem is, both extractions are highly energy-intensive. They are not feasible in a world of comparative energy scarcity.
Another approach is to burn jet fuel distilled from conventionally-obtained oil, just as we do today, but to offset all of the carbon emitting into the atmosphere with carbon extracted from the atmosphere, through ‘direct air capture.’ Today, facilities like Climeworks' Orca plant in Iceland can extract CO₂ at a cost of approximately $600-to-$800 per ton. That price has fallen from roughly $2,000 per ton in 2015, and as deployment scales, is projected to reach $100-to-$200 per ton by 2030.
That will still not be enough. A Fermi estimate suggests that replacing all the jet fuel burned in the world today with sustainable aviation fuel would require 10,000 terawatt-hours (TWh) of electricity. If we imagine a future world where everyone flies twice as often as they do now, 20,000 TWh of electricity would be needed; that amount is four times the amount of electricity that the United States produces in its entirety today, or about 70% of the world’s total production.
The challenge presented is daunting. But, as Too Cheap to Meter demonstrates, the creation of a solar industry seemed daunting as well; but once production of solar panels began, learning curves in production rapidly brought costs down. Pursuing the production of sustainable aviation fuel and new sources of energy today will do the same, making sustainable jet fuel, direct air capture, and abundant air travel all possible.
But it will only be possible if we accept that the right approach is not to do less, but to do more.
Hyper Abundance
Energy access is a fundamental enabler of human capability and dignity. In Too Cheap to Meter, Doug Bernauer says “Anyone who thinks that the limitation of energy is a good idea is totally ignorant as to the role that energy plays in everyday life.” Human flourishing depends fundamentally on energy abundance. For billions still seeking to escape poverty, this abundance is a necessity.
But what lies beyond mere sufficiency? What becomes possible when we achieve not just energy abundance, but hyper-abundance? The final act of Too Cheap to Meter ventures into this territory, envisioning a future where energy constraints effectively disappear.
With virtually unlimited clean energy, we could finally address our most intractable global challenges. Water scarcity, which affects billions today, would become nothing more than a mundane engineering problem. Large-scale desalination, currently limited by energy costs, could provide fresh water wherever needed. We could restore old lakes and seas, transforming arid regions into fertile landscapes and ending the water competition that drives conflict in so many parts of the world.
Climate repair moves from theoretical possibility to practical reality in a hyper-abundant energy future. Direct air capture of carbon dioxide—technology that, like desalination, exists today but remains prohibitively energy-intensive—could be deployed at planetary scale. “With enough energy,” as Isaiah Taylor notes in the film, “we can remove carbon from the atmosphere” and effectively reverse centuries of industrial emissions.
Rather than merely slowing damage, we could actively heal our climate system.
Perhaps most profound is the expansion of humanity's physical horizons. Space exploration and colonization, long constrained by the immense energy requirements of escaping Earth's gravity well, would finally become feasible. The possibility of becoming a multi-planetary species—establishing self-sustaining colonies on Mars and eventually beyond—represents not just an insurance policy against terrestrial catastrophe but an expansion of human potential.
"The next step," says Taylor, “is to be among the stars.” As he discusses in Too Cheap to Meter, O'Neill cylinders—vast rotating habitats in space providing Earth-like living conditions—are one feature of such an expansive future. “If you can create one O'Neill cylinder, you can create thousands.” Such habitats would represent not just technological marvels but new frontiers for human civilization, limited only by our imagination and the materials available in our solar system.
More immediate applications would transform life on Earth. Hyper-abundant energy would enable us to rethink the design of our cities, transportation systems, and industrial processes:
Vertical farming at massive scale could return vast tracts of agricultural land to nature
Universal electrification and automated manufacturing could eliminate drudgery while creating unprecedented material prosperity
AI and computing capabilities could expand dramatically
Too Cheap to Meter suggests that computation might be the ultimate expression of energy hyper-abundance, allowing the creation and training of AI models that could solve our most complex problems, from disease to climate modeling.
This vision of energy hyper-abundance is, today, a vision to inspire us, not a blueprint of our immediate next steps. But it is inspiring because it is not idle fantasy. It is a distant, but logical extrapolation of the human journey. Each transition to more concentrated energy has expanded what's possible, and it’s reasonable to suppose that energy abundance in our time could lead to hyper-abundance in the future.
We stand at an inflection point. We can, if we choose, embrace the potential of energy abundance. If we take this path, we commit ourselves not to choosing one perfect energy source, but instead to attempt to accelerate progress on all sources simultaneously. By advancing solar, fission, fusion, and every other promising technology, we can create a portfolio of solutions, to ensure that for every situation and circumstance we have the right tool to hand.
Energy abundance represents humanity's greatest opportunity: the key to unlock solutions to challenges we've struggled with for generations. From ending poverty to healing our climate, from building new things in space while rebuilding the Earth, abundant energy can be the foundation upon which we can build a truly expansive civilization.
The first step is to recognize that the future requires us to innovate. Our task is not to live with less energy, but produce vastly more of it.
Thank you for reading, watching, and supporting our work at Story Co.